Lattice Quantum Chromodynamics demystified
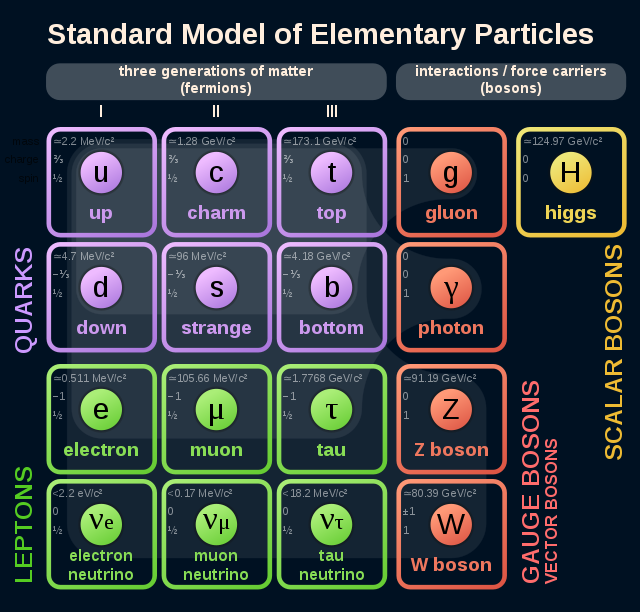
Hello everyone. It’s been a while since my previous post since PRACE SoHPC kept me busy! Before introducing my project to you, I would like to explain some basic concepts to clarify in advance some ambiguous scientific stuff you are going to come across by following my posts. So grab your favourite snack or beverage and let me talk about some cool physics concepts!
Particle Physics Basics
All the existent particles are divided into elementary particles and composite particles. The former ones are not comprised of other particles while the latter ones are. All charged particles are considered to have an antiparticle that has the same properties but an opposite charge. In order to classify the elementary particles based on their properties and introduce the rules of their interaction, we resolve to a theory called the Standard Model. This theory divides them into fermions and bosons depending on the particle property called spin. Fermions have a half-integer spin and obey the Pauli exclusion principle. In contrast, bosons have integer spin and don’t obey the Pauli exclusion principle.
The bosons are what we call force mediators. This means, that every particle interaction can be viewed as an exchange of a boson. The only exception is the newly discovered Higgs boson whose field is associated with giving other particles their inertial mass. Other than that, the remaining bosons are the photon, the gluon, and the W & Z bosons which are the carriers of the electromagnetic, the strong, and the weak force respectively. Moreover, each of those forces has an associated charge which particles must have in order to participate in that interaction. Therefore, there is the electric charge for the electromagnetic force, the colour charge for the strong force, and the weak charge for the weak force. An interesting fact is that, unlike electrically neutral photons, gluons and W & Z bosons carry the charge corresponding to the force they mediate. As a result, they can interact with themselves.
The fermions are the particles responsible for making up the matter and are in turn separated into quarks and leptons. Their main difference is that quarks have colour charge while leptons do not. However, both of them come up in six flavors that can be categorised into three generations, where particles in different generations have similar properties but different masses. Specifically, the existent quarks are: down, up, strange, charm, bottom, and top. The existent leptons are: electron, muon, tau, electron neutrino, muon neutrino, and tau neutrino. All those particles except for the neutrinos have an electric charge.
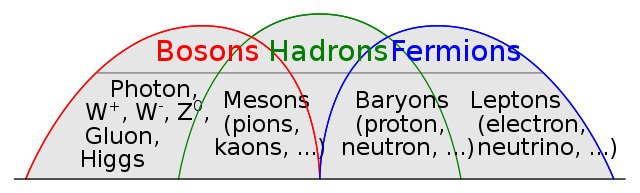
Since we got introduced to the elementary particles, we should also make a quick reference to the composite particles. Even though they consist of hadrons, atomic nuclei, atoms, and molecules, we will be mainly interested in hadrons since the rest are a combination of hadrons and elementary particles. Hadrons are made of quarks and are divided into baryons, which have an odd number of quarks(usually three quarks), and mesons, which are made of an even number of quarks(usually one quark and one antiquark) and are relatively unstable. Due to the spin resulting from their number of quarks, baryons are fermions while mesons are bosons. The most popular baryons are protons and neutrons whereas the most popular mesons are pions and kaons.
What is Lattice Quantum Chromodynamics?
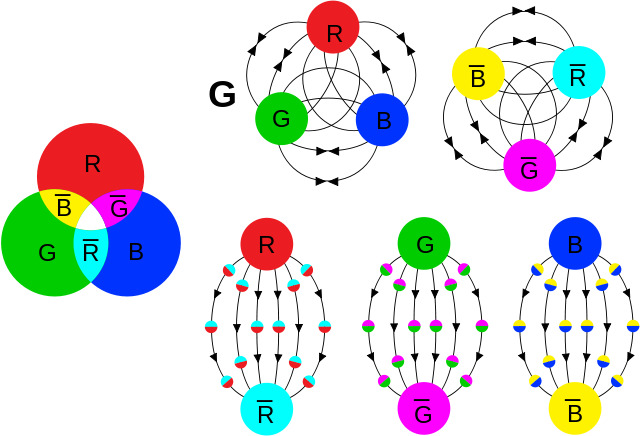
In the above section, we mentioned a new type of charge called colour charge and associated it with the strong force. Initially, we should clarify what exactly the strong force does. As we discussed, quarks are the main constituents of matter since they form the protons and neutrons, while gluons mediate the strong force just like photons mediate the electromagnetic force. However, one should wonder how quarks are bound together since their small mass could enable them to move constantly at speeds close to the speed of light. The answer to this question is that they are held together by the strong force, which is indeed the strongest of the four forces!
Now that we have understood the strong force, we need to shed some light on the colour charge. This charge has three colours red, green, blue, and three anticolours. A particle made up of all three colours or anticolours or one colour and its corresponding anticolour has a neutral colour and electric charge. The colour charge can also explain the existence of some composite particles such as the delta baryon which is constructed by the combination of three up or down quarks. Without the property of the colour charge, there would be two quarks with the same spin, which would violate the Pauli exclusion principle. Consequently, just like Quantum Electrodynamics(QED) is used to explain electromagnetic interactions, there is the theory of Quantum Chromodynamics(QCD) which describes the action of the strong force.
In physics problems, it is quite common to rely on what is referred to as perturbative solutions. This entails finding an approximate solution to a problem, by starting from the exact solution of a related, simpler problem. For instance, you can think of the Taylor series expansion of a challenging function. That would be a great way to solve QCD problems but there’s a catch. The highly nonlinear nature of the strong force and the large coupling constant at low energies makes finding perturbative solutions extremely complicated. For this reason, physicists tend to formulate QCD in discrete rather than in continuous spacetime by using a lattice. Lattice QCD(LQCD) is a non-perturbative approach that simplifies the daunting task of solving QCD problems.
What is my goal with the project?
To conclude our extensive discussion about the fascinating world of LQCD, it is about time that I describe my project’s objective. That is to efficiently use a tool called chromaform with the help of HPC resources and make several QCD simulations. The target of them is to extract successfully the mass of a pion and then find an input quark mass that would result in a pion mass close to the experimental one. Those experiments will give us a good idea of how much the input quark mass and some omitted quark-loop effects influence the resulting pion mass. In my next blog post, I will explain the whole process much more thoroughly and provide my results in its step.